
These two impedance matching mechanisms effectively transmit air-born sound into the fluid of the inner ear. Also because the arm of the malleus to which the tympanic membrane is attached is longer than the arm of the incus to which the stapes is attached, there is a slight amplification of the sound pressure by a lever action. Notice that the round window membrane, located beneath the oval window, moves in the opposite direction.īecause the tympanic membrane has a larger area than the stapes footplate there is a hydraulic amplification of the sound pressure. Since fluid is incompressible, as the stapes moves in and out there needs to be a compensatory movement in the opposite direction. The footplate of the stapes fits tightly into the oval window of the bony cochlea. The resulting movements of the eardrum are transmitted through the three middle-ear ossicles (malleus, incus and stapes) to the fluid of the inner ear. The pinna and external auditory meatus collect these waves, change them slightly, and direct them to the tympanic membrane. Obviously, if we heard that ceaseless (except at absolute zero) motion of air molecules we would have no silence.Īir-conducted sounds eventually move the inner-ear fluid.įigure 12.2 depicts these alternating compression and rarefaction (pressure) waves impinging on the ear. Humans can detect sounds that cause movements only slightly greater than those of Brownian movement. Sounds often vary in frequency and intensity over time.
#AUDITORY CANAL FUNCTION SERIES#
Sound is a series of pressure changes in the air. The auditory system changes a wide range of weak mechanical signals into a complex series of electrical signals in the central nervous system. Bending the cilia to the side has no effect on spontaneous neural activity.ġ2.2 Sound: Intensity, Frequency, Outer and Middle Ear Mechanisms, Impedance Matching by Area and Lever Ratios Bending the cilia in the opposite direction closes the channels and decreases afferent activity. Bending the cilia toward the tallest one opens the potassium channels and increases afferent activity. Hair cells normally have a small influx of K + at rest, so there is some baseline activity in the afferent neurons. Press the "play" button to see the mechanical-to-electrical transduction. This in turn causes neurotransmitter release at the basal end of the hair cell, eliciting an action potential in the dendrites of the VIIIth cranial nerve. An inward K + current depolarizes the cell, and opens voltage-dependent calcium channels. This mechanism transduces mechanical energy into neural impulses. Opening these channels allows an influx of potassium, which in turns opens calcium channels that initiates the receptor potential. When the cilia are bent toward the tallest one, the channels are opened, much like a trap door. The tip links function like a string connected to a hinged hatch. There are tiny thread-like connections from the tip of each cilium to a non-specific cation channel on the side of the taller neighboring cilium. These cilia increase in length along a consistent axis. Cilia emerge from the apical surface of hair cells. Tight junctions between hair cells and the nearby supporting cells form a barrier between endolymph and perilymph that maintains the ionic imbalance.įigure 12.1 illustrates the process of mechanical transduction at the tips of the hair cell cilia. This actively maintained ionic imbalance provides an energy store, which is used to trigger neural action potentials when the hair cells are moved. The fluid, termed endolymph, which surrounds the hair cells is rich in potassium.

(See the chapter on Vestibular System: Structure and Function). Hair cells in the maculae of the saccule and the utricle respond to linear acceleration (gravity). Hair cells in the cristae ampullares in the semicircular ducts respond to angular acceleration (rotation of the head).
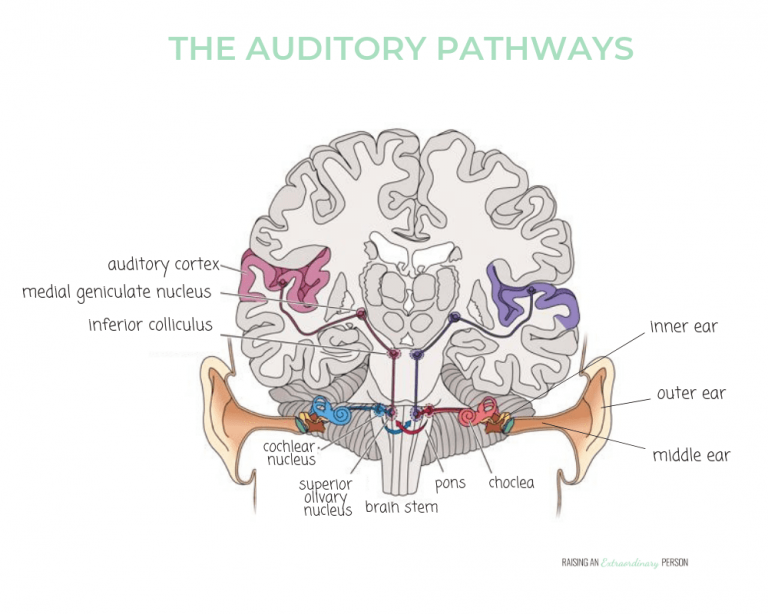
Hair cells in the Organ of Corti in the cochlea of the ear respond to sound. Several specializations make human hair cells responsive to various forms of mechanical stimulation. In higher vertebrates the internal fluid of the inner ear (not external fluid as in fish) bathes the hair cells, but these cells still sense movements in the surrounding fluid. The hair cell first appeared in fish as part of a long, thin array along the side of the body, sensing movements in the water. The key structure in the vertebrate auditory and vestibular systems is the hair cell.
